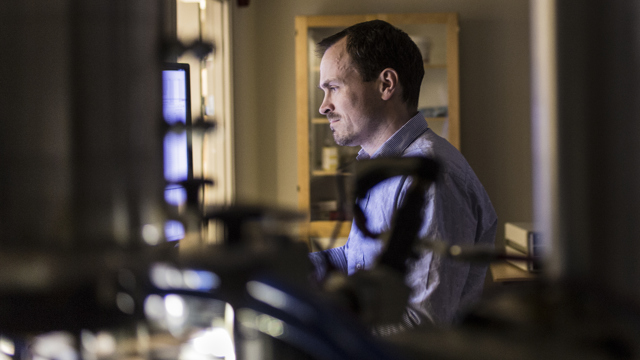
Quick development
Development is moving quickly: if you need a spare part for your car, in the future you could perhaps go to the factory, which will quickly print out the part you need from its saved CAD blueprints for your car model. Neither warehouse nor machinery needed. There are factories in every neighborhood.Most is possible in theory, but how does it look in practice?

In the steel industry, there is a great deal of knowledge about high-strength steel, and in the processing industry there is knowledge of how the steel can best be processed, but when it’s a question of additive manufacturing, everything – and then some – is needed all at once.
“In advance products, such as airplanes, we have to know exactly what properties the material we’re putting into the products has,” adds Magnus Kahlin, industrial doctoral student in the division.
He works at Saab, and this doctoral student project deals with studying the properties in materials such as aluminium and titanium – both important to the aviation industry.
Advantages
The advantages of additive manufacturing are obvious; one small design change today means not only a new blueprint, but also that new tools need to be developed – a cost of several millions.
“But the industry wants robust processes; the material properties cannot vary other than within a very small span when a small detail is changed, a new machine is bought, or the powder is recycled,” he explains.
The research group is now studying additive technologies that involve metal powder being spread out in layer upon thin layer, in which each layer is fused together with the previous one using lasers or an electron beam. A 3D blueprint in the computer governs the beam; the metal is fused together only where the beam hits its mark, while the powder around it and in any cavities remains powder. This is gathered together and used for the next printing, which means that material wastage becomes minimal.
Nor as regards additive manufacturing are the powder or the metal the major cost, but the construction time itself. The powder costs approximately as much regardless of what metal it is, which also changes the economy of manufacture.
Mr Kahlin is studying the microstructure of titanium and aluminium in detail under an electron microscope. Small test rods are exposed to both static and dynamic stresses until they break and compared with equivalent rolled or extruded details (the metal is pressed or sprayed through a form). Dunyong Deng, also a doctoral student, will instead study the high-strength nickel alloys that are very common, in gas turbines for example. In his project, he will also compare welded and forged details with additive manufacturing in the same alloy.
“We’re beginning to understand how the microstructure affects the formation of cracks in the conventional material. We’ll now be looking at the same material in additive manufacturing. Based on the
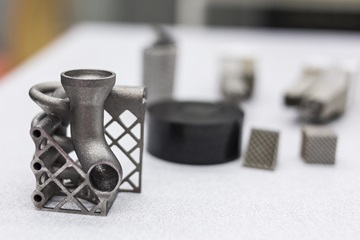
Heat-treated materials
But so far they are only suspicions; the research will provide answers.“We’re also studying metals that often need to be heat-treated in complicated processes to get the properties the industry is trying to obtain. The additively manufacture material probably behaves in a different way; at a guess, entirely different processes will be needed,” he says.
The funding is coming from several different directions: the Swedish Foundation for Strategic Research (SSF), for example, is funding Mr Kahlin’s industrial doctoral student job along with Saab. Mr Deng is conducting his doctoral student studies on a scholarship from his homeland, China, and money is also coming from the strategic investment in advanced functional materials (AFM) at LiU, which receives annual grants from SSF. Yet another doctoral student is linked to the group: Jonas Saarimäki, who is researching the mechanisms of crack propagation in nickel-based materials for turbines.
“There are, naturally, several research groups in Sweden and the world who are looking more closely at additively manufactured materials, but certain properties are very product-like and we’ll try to imitate the damage mechanisms that exist in the industry as much as possible,” Professor Moverare says.
Additive manufacturing
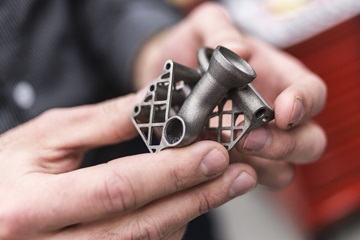
An object or product is produced by a 3D model in the computer being divided up into very thin layers, and the layers are built on one another until the object is finished. The material varies; there are machines that print in gypsum, plaster, metals, and biological materials.
The technology is currently used industrially, where completed products are manufactured, for example, in titanium, aluminium, and various plastic materials.
Products manufactured in metal have been on the market for more than ten years. Two manufacturing technologies predominate: fusion with lasers, or Selective Laser Melting (SLM) and fusion with an electron beam, or Electron Beam Melting (EBM). The Swedish company Arcam is one of the leaders in the field with its EBM machines, which are used both for orthopedic implants and for spare parts production.
The inkjet technique is also used especially for manufacture in biological materials and various polymers.
Researchers at LiU are studying materials from various types of machines that construct from metal powders such as titanium, nickel alloys, and steel. The object studied come from several different directions, and they are manufactured in industry-like products.